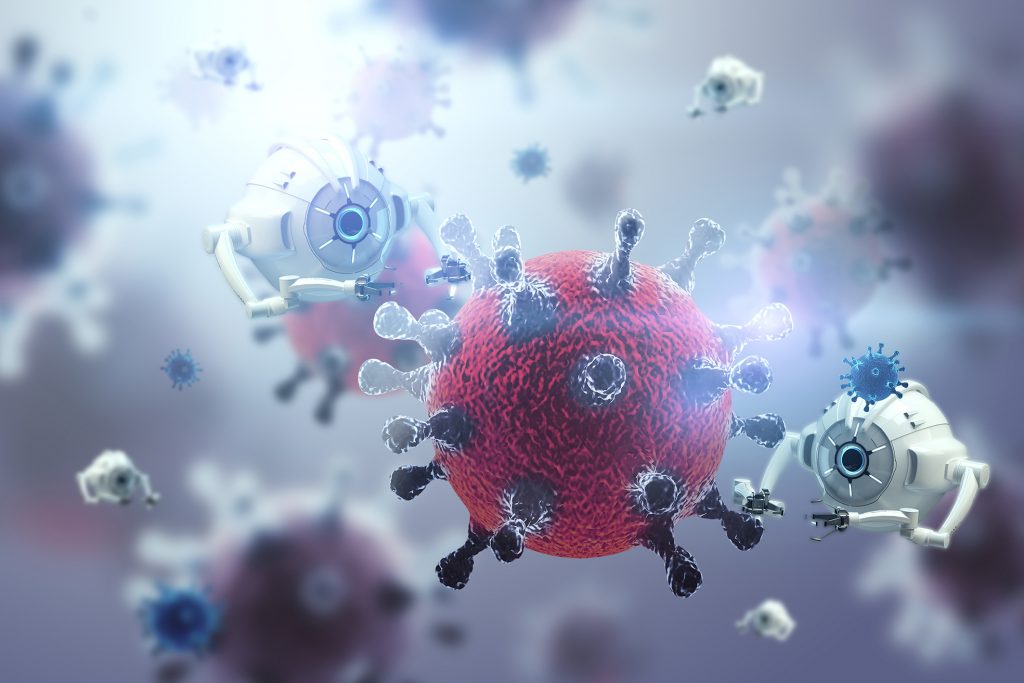
The COVID-19 pandemic has had an indelible effect, with at least 517,779,437 cases and 6,278,165 related deaths reported globally.1 Despite substantial multi-disciplinary research efforts to combat SARS-CoV-2, there is a crucial need to revolutionize current diagnostic and management approaches in preparation for emerging variants of concern. Nanotechnology has significant potential to design new approaches and improve existing antiviral strategies, by facilitating the design and development of:2
- Containment-safe personal protective equipment (PPE) to enhance the safety of frontline personnel, and anti-viral disinfectants and surface coatings to inactivate the virus and prevent its spread.
- Highly specific and sensitive nano-based sensors (nanodiagnostics) to quickly identify the infection or immunological response.
- New drugs (nanotherapeutics) with enhanced activity, decreased toxicity, sustained release, and tissue-targeting properties.
- Nano-based vaccination (nanovaccines) to boost humoral and cellular immune responses.
Current PPE systems are limited because filter media is unable to inactivate microbes or prevent their spread. An array of nanotechnology-based modalities have been explored, employing varied nanoparticles (NPs), both to suppress viral transmission and to develop antiviral coatings that can diminish the adhesion of SARS-CoV-2.
Recent findings and developments include:3
- The deposition of antiviral metal NPs such as AgNPs and AuNPs on filter surfaces may enhance the efficacy of surgical masks and respirators.
- Multifunctional metallic ‘nanoflowers’ with unique air stability and substrate-adherence properties can inactivate pathogens by disrupting their cellular functions, and can be formulated for use as sprays (aerosols) or for deposition on masks.
- TorStran, a novel virus filter made of a thin carbon nanotube mat (with improved air permeability and filtration properties) may capture and instantly deactivate airborne/aerosolized droplet viruses.
- Polyvinylidene fluoride (PVDF) electret nanofibers can capture over 90% facsimiles of airborne COVID-19 particles.
- A highly breathable nanofiber-based cellulose, for use in biodegradable, anti-pollution masks as a disposable filter cartridge, may assist long-duration mask-wearers and those with existing respiratory conditions.
Nanodiagnostic strategies are underway for effective detection, surveillance, and monitoring of SARS-CoV-2, addressing key drawbacks of widely employed RT-PCR (real-time polymerase chain reaction) and quantitative (qRT-PCR) methods of diagnosis – false negative/false positive outcomes, cost- and time- constraints, and the need for technical expertise.
Recent strategies include:3,4
- Point-of-care testing methods using Ag/AuNPs for the effective detection of human anti-SARS-CoV-2 IgG and/or IgM.
- ‘Respi-strip’, an immunochromatographic assay, was built using a membrane with colloidal AuNPs for testing nasopharyngeal specimens from symptomatic patients. The assay served as an essential rapid diagnostic test with 99.5% specificity and required approximately 15 minutes of testing time, thus facilitating early care and isolation of COVID-positive patients.
- A lateral flow immunoassay (LFIA) was developed using lanthanide-doped polystyrene NPs to detect the presence of anti-SARS-CoV-2 in human serum. The assay could be completed in 10 minutes, and results were comparable to those of RT-PCR.
- Magnetic NPs were used to devise a simple, highly sensitive detection method using RNA extraction.
- Plasmonic chips using gold nanoislands provided precise detection of the nucleic acid of SARS-CoV-2, with a lower detection limit of up to 0.22 pM.
- A colorimetric dual-mode LFIA using quantum dots (QDs) nanobeads enabled qualitative measurement of anti-SARS-CoV-2 IgM/IgG, resulting in 100% sensitivity and specificity of a SARS-CoV-2 diagnosis.
Lastly, nanotechnology-based techniques can also contribute to the next generation of approaches to COVID-19 treatment and prevention:3-6
- NPs of different designs and compositions, namely virus-like particles (VLPs), polymeric NPs, self-assembled protein structures, QDs, and inorganic NPs, have been utilized for the preparation of COVID-19 vaccines.
- Current lipid NP-based mRNA vaccines serve as significant frontrunners for SARS-CoV-2 prevention.
- Nanovaccines have the potential to provide high mucosal immunity in the lungs (inhalational vaccines), along with enhanced safety and convenience; furthermore, nanovaccines have the potential to elicit both systemic and local immune responses (intranasal vaccines) to combat respiratory infections.6
- Antiviral AuNPs are effective in suppressing virus entry into the target cell by interacting with and oxidizing disulfide bonds in viral
- Stimuli-responsive drug delivery systems have the potential to target pathogens and infected cells and administer the drug across biological barriers.
- NP carriers were found to enhance patient compliance by rendering controlled/sustained drug release capabilities.
- An oral nanomicelle formulation of curcumin, when administrated in COVID-19 patients, aided in faster elimination of symptoms. Likewise, niclosamide-loaded lipid NPs suppressed SARS-CoV-2 replication in vitro.
NP-based interventions could be instrumental to counteracting the transmissibility and virulence of SARS-CoV-2, while nanostructured drug discovery and delivery systems have great potential in SARS-CoV-2 treatment and prevention. With further research, nanotechnology could play a key role in halting the ongoing pandemic and enhance our preparedness for future disease outbreaks.
References
- COVID-19 Coronavirus Pandemic 2020, Available from: https://www.worldometers.info/coronavirus/. Accessed 10 May 2022.
- Campos EVR, et al. J Nanobiotechnol 2020;18:125.
- Pandey A, et al. ACS Biomater Sci Eng2021; 7:31–54.
- Derakhshan MA, Amani A, Faridi-Majidi R. ACS Appl Mater Interfaces 2021; 13:14816-14843.
- Hasanzadeh A, et al. Journal of controlled release 2021;336:354–374.
- Yayehrad AT, et al. Int J Nanomedicine 2021;16:5713-5743.